From its first use in studying the photoelectric effect, electron microscopy has come a long way, penetrating research workflows and expanding its reach into every facet of the scientific community. Its applications are no longer confined to the material science research questions EM had its humble beginnings in. We see researchers use electron microscopes in studying fibers in clothing, blood samples, and gunshot residue for forensic research just as much as we see EM used as a tool for the exploration of disease-fighting drug development pipelines and solar cell research. As research toward a healthier, greener future is spurred forward, electron microscopes position themselves at the forefront of these undertakings as the ultimate tool for studying the nanoworld- where the signs of all future implications first start taking form.
What does such a nanoworld look like? What inferences can be drawn from the seemingly minuscule changes that occur in this world teeming with moving, interacting three-dimensional structures? These questions drive electron microscopists forward in their analyses every day. In such an attempt at understanding the nanoworld, we at Delmic set out to interview leading EM scientists, so we could envisage how life & matter unfurls at the nanoscale through their perspectives. Here’s what they had to say!
On EM Zen and discovering the beauty of life
Lucy Collinson 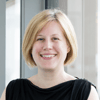
Head of Electron Microscopy, The Francis Crick Institute
In the early days, when I was still in the lab embedding samples and imaging them, EM taught me to slow down and expect things not to work the first time (or the second or third time!). I now call this state of mind ‘EM Zen’. It taught me to put a limit on the amount of time I think about something before I go ahead and try it because it’s only then that you know if something will work. EM also taught me to logically troubleshoot – to decide and prioritize the most likely cause of a problem and then change one thing at a time until it works. It inspired me by revealing the complexity and beauty of life at the smallest scales, but then made me realize that if you get too caught up in the details, you miss the complexity and the beauty of the big picture. And it has shown me that when a community works collaboratively rather than competitively, you can achieve great things in a very short space of time.
Life, a 3-D machinery of molecular trafficking!
Zach Freyberg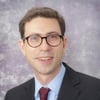
Associate Professor, Departments of Psychiatry and Cell Biology, University of Pittsburgh
For as long as I can remember, I have been curious about the behavior of cells, and, more specifically, how cells traffick proteins in healthy versus diseased states. As a cell biologist, this led me to the study of cell organelles, and how they shape the processes by which proteins get synthesized and trafficked to their intended destinations. As a physician, these questions are equally intriguing. Indeed, when these cellular trafficking mechanisms sputter or fail, proteins begin accumulating in cells throughout the body and can lead to profound disease states as in Parkinson’s disease or Alzheimer’s disease. Being a visual person, I wanted to be able to picture these complex cellular events and have spent my career trying to get closer and closer to actually seeing the machinery of trafficking in fine molecular detail.
Moreover, I wanted to directly visualize what goes wrong in disease in the cells of patients in similarly fine detail – to get new insights into molecular mechanisms not only biochemically, but directly in cells in contexts where they matter. This led me to electron microscopy. During graduate school, I started probing these questions of trafficking by conventional two-dimensional EM, focusing on the Golgi apparatus and the contributions of specific species of lipids to Golgi structure and its roles in cell trafficking. This work revealed the fine convolutions of intricate stacks of membranes which were not only visually appealing but that yielded important new functional information.
This planted the seed of an idea – that the shape of cellular organelle has something to do with its function. Such an idea ultimately led me to new forms of EM, namely cryo-electron tomography, or cryo-ET. Here, I could look into cells in near-native states and not just in two dimensions, but in three! In fact, cryo-ET of secretory cells revealed a much more complex state of things than I ever imagined. In some of the very first experiments, my collaborators and I were able to visualize a new form of the endoplasmic reticulum that had a vesicular shape – something that was definitely not in the textbooks! This led to a whole series of studies that characterized this curious vesicular organelle and its relevance to local protein synthesis – a process that has long been studied but is still not well understood structurally. Moreover, this ability to visualize the cell’s organelles in 3D changed the way I look at disease. It got me to ask new questions about the cellular nature of disease states. Does disease occur, at least some of the time, when the structures within an organelle, specific protein complexes, change their shape due to mutations or cell stress, for example? And do these changes in protein shape alter the structures of the organelles and how they function? In a sense, these are architecture questions – does form define function? These basic questions now drive a lot of my research and the way that I apply EM to study cell biology.
Exploring the frontiers of quantum mechanics
Nahid Talebi 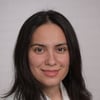
Professor, Institute for Experimental and Applied Physics, Christian Albrechts University in Kiel
During the last year of my PhD studies in 2011, I visited the Max Planck Institute in Stuttgart to realize a nonlinear optics circuit. During this time, I collaborated with the Stuttgart Center for Electron Microscopy to simulate the plasmonic response of simple nanostructures. I immediately became fascinated by the fundamentals of electron-light interactions, which could be mediated by the near-field zone of nanostructures. The ability of electron microscopes to control single-moving quantum objects, i.e., electron wave packets, and let them interact with nanomaterials, for imaging and spectroscopy, provides a toolbox to control single-electron and single-photon interactions. This allows us to fully explore the frontiers of quantum mechanics - a fact that I am still fully excited about it – different from the interaction of coherent (electrodynamic classical) laser light with matter. By getting to know the concept of photon-induced near-field electron microscopy, yet another simple idea came to my mind: Could we not let the electrons generate their own light, instead of using lasers? Electron beams are anyway used as sources of light, by means of various processes such as Cherenkov or Smith-Purcell radiation. Merging such radiation sources with electron microscopes could allow for multi-sequential interactions with the advantage of exploring coherent and decoherence phenomena and photon-assisted electron-beam spectroscopy. This is still a research direction I pursue. Hence, electron microscopes have paved my path to research in a non-classical way.
Visualizing diseased tissue in unprecedented detail
Gerald Shami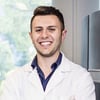
Multimodal Electron Microscopist, Australian Centre for Microscopy & Microanalysis (ACMM)
Characterizing structure-function relationships is fundamental to answering questions in cell biology, with a common objective of understanding disease processes and thus improving clinical outcomes for patients. No other biomedical imaging technique has contributed more to this endeavor than electron microscopy (EM). EM provides an unprecedented and holistic view of the entire spectrum and scale amongst which biological organisms and their comprising constituents exist.
In my own work, we have utilized multimodal electron microscopy including array tomography, thin-section TEM and electron tomography in order to characterize giant mitochondria in patients afflicted by non-alcoholic fatty liver disease (NAFLD). EM has allowed us to observe structural changes, resulting in a loss of normal mitochondrial function. By harnessing large-volume EM, we could also visualize and quantify the entire chondriome (mitochondrial population) in these patients, thereby complementing our structural observations with rigorous morphometric information and further proposing a mechanism of the formation of these anomalous structures. Thus, electron microscopy has allowed us to tangibly visualize the structure of normal and diseased tissues at a level of detail not conferred by any other technique used within the biological sciences.
Pursuing research topics previously deemed unimaginable
Benjamin Lawrie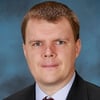
Research Scientist, Oakridge National Laboratory
Many of the most exciting basic research opportunities are increasingly interdisciplinary. My research interests at Oak Ridge National Laboratory were initially focused on quantum optics and quantum sensing, but I had the opportunity to collaborate with ORNL’s Scanning Transmission Electron Microscopy Group on some nascent cathodoluminescence experiments a few years after I arrived at the lab. As a result of those early collaborations, and with support from ORNL’s Center for Nanophase Materials Sciences, we were able to develop new research efforts targeting in situ optical and electron-beam manipulation of color centers and excitons in nanoscale and 2D materials using a Delmic SPARC cathodoluminescence detector with a Thermo Fisher Quattro SEM.
I still lean heavily on internal and external collaborators to help develop new measurement modalities, and my research interests have continued to evolve because of those collaborations. We’ve developed new approaches to control quantum light sources through these collaborations, but we’ve also developed new multimodal probes of optoelectronic films and devices that serve as the foundation of emerging microelectronic technologies, a research topic I never would have imagined pursuing at the start of my career.
Demystifying the greatest of mysteries: life
Stefan Raunser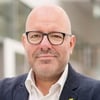
Director, Dep. Structural Biochemistry, Max Planck Institute of Molecular Physiology
Electron microscopy, especially electron cryomicroscopy (cryo-EM), has been the gateway to many discoveries in my academic life for more than 22 years. Cryo-EM reveals a spectacular universe of form and structure we would never otherwise have access to. The first time peeking through an electron microscope at the start of my Ph.D. thesis with Werner Kühlbrandt at MPI of Biophysics in Frankfurt is a moment I shall never forget: what I saw were strikingly beautiful two-dimensional protein crystals and I realized that with the electron microscope, you can explore an otherwise hidden world. Immensely moved by my initial experience, I embarked on a long journey of scientific devotion to single-particle cryo-EM as well as electron cryotomography (cryo-ET).
Meticulously working with cryo-EM and cryo-ET on a daily basis for two decades has changed the way I see the world. One of the most prominent ways for us scientists to understand the mysteries of the universe is through direct observation, relying heavily on our ability to scale everything visually. Hence, I find myself constantly thinking in a visuospatial manner regardless of whether I am reading a scientific article or listening to a lecture. I close my eyes and can immediately sketch out the possibilities of how molecules may interact in space. Cryo-EM, which rightfully won the Nobel prize in 2017, offers an infinite world of possibilities due to its advanced technology and allows one to tackle complex problems previously thought impossible. Our mission in this field is clear: to map out all living organisms no matter how minuscule and become closer to solving the greatest mystery of the universe: life.
In conclusion, electron microscopy helps you break the barriers of research, bringing you one step closer to visualizing our world at the nanoscale. How has EM shaped your research paths and the way you think?